The pandemic has changed nearly all of our daily rhythms and yearly traditions but it hasn’t erased them altogether. Instead of our annual holiday party, we had an outdoor fall lab BBQ with fire pit and s’mores. While the masks were different, the good food and good company was the same.
The holiday party has become a fun tradition in the lab. We eat lots of food, do a white elephant gift exchange, play board games, take a new group picture, and toast to a new year.
I had a wonderful opportunity to visit Zheliang University in Hangzhou, China, where Rui Xiao and Zheng Jia are now professors. They organized a wonderful visit for me, capped with a joint group meeting of their students. They each presented their research projects. I was impressed by the depth and creativity of their works. It’s wonderful to see how well Zheng and Rui are both doing in their career.
We had an impromptu lunch catching up with current and former lab members at the Summer Biomechanics, Bioengineering and Biotransport Conference at Seven Springs, PA. Sara Bentil is 3 years into her Assistant Professor position at Iowa State and attending her first SB3C. Kristin Myer is newly tenured at Columbia and returning from a sabbatical and Dan Midgett is doing new and cool things at in biomechanics and cardiology at Yale. Along with Arina Korneva and Tracy Ling, it was a great group.
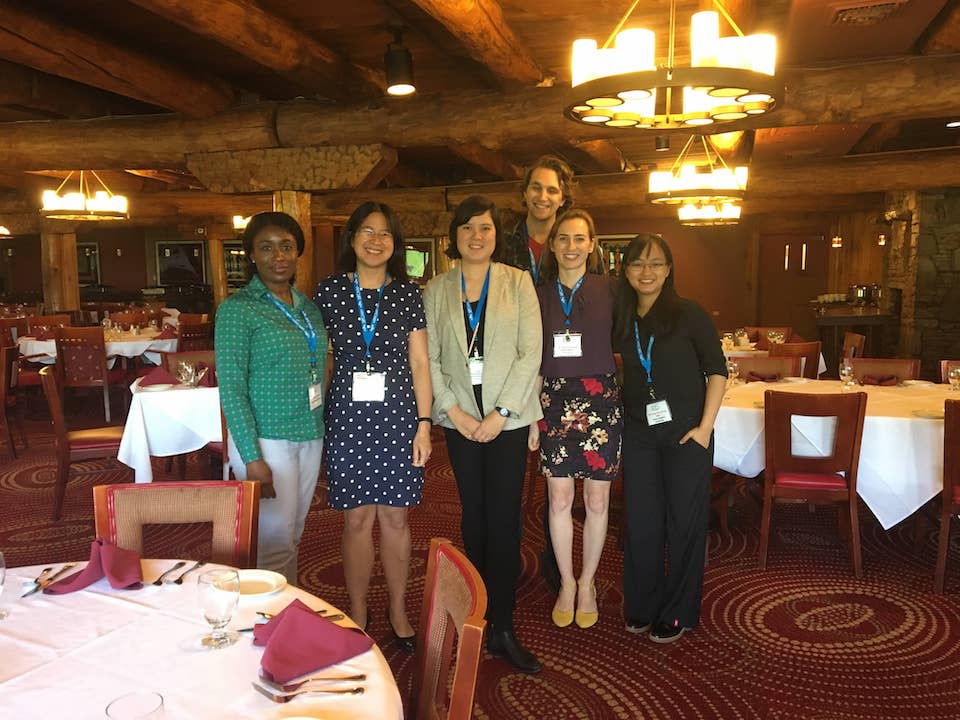
Congratulations to Dr. Jiayu Liu for his successful dissertation defense, “Mechanics-Based Design of Stimuli-Responsive Hydrogel Structures and Devices“.
Abstract: Stimuli-responsive hydrogels undergo large swelling in response to a wide variety of stimuli, such as temperature, biochemical molecule, pH, electric or magnetic field. Programmable shape changing devices made from stimuli-responsive hydrogels have potentially wide-ranging applications, including drug delivery, soft robotics, and biomedical devices. The current main challenges of advancing stimuli-responsive hydrogel devices into real-life applications are in both the design and fabrication stage. Simple strategies based on mechanics are needed to guide the design of hydrogel devices with complicated shape changes. Traditional fabrication methods such as 2D lithography limits the number of achievable device geometries. The objective of this work is to develop a methodology that covers from constitutive modeling, finite element simulation, mechanics-based design rules, to experimental validation. This hybrid modeling design methodology provides an efficient design framework for stimuli responsive hydrogel structures and devices of functional importance. In the first part of this work, we applied a chemo-mechanical constitutive model to describe the swelling and mechanical behavior of a novel DNA hydrogel (AAm-co-DNA). In order to estimate the elastic modulus of DNA hydrogel that cannot be measured by traditional experimental approach, we used finite element analysis to study the curving of bilayer beams composed of the DNA gel and a passive gel (polyacrylamide, pAAM), and compared the finite element simulations to the experiment result. We further applied the finite element model to investigate the influence of bilayer geometric and material properties on the equilibrium curvature. We used the modified Stoney formula for the curving of film/substrate system to develop a simple design rule for predicting the equilibrium curvature of bilayer gel beams with different dimensions and material properties. In the second part of this work, we report the design, fabrication, and characterization of segmented 3D printed gel tubes composed of an active thermally responsive swelling gel (poly N-isopropylacrylamide, pNIPAM) and a passive thermally nonresponsive gel (pAAM). Using finite element simulations and experiments, we report a variety of primitive shape changes including uniaxial elongation, radial expansion, bending, and gripping based on different segment arrangements of two gels. The assembly of shape-changing primitives could be directly printed and used to achieve complicated tasks. In the final part of this work, we report on the unusual periodic buckling behavior of a 4D printed tubular structure, composed of alternating vertical strips of pNIPAM segments and pAAM segments. The tube design was inspired by the buckled surfaces observed in nature, such as on cacti and euphorbias. We found that the tubes show tunable periodic buckling modes in water at the room temperature, due to the development of compressive stresses in the soft swellable segments induced by the constraint of the stiff non-swellable segments. We developed finite element models to explore the design space and investigate the effects of geometric and material design parameters on the buckling mode. Modeling the swellable segments as the buckling of a bar on an elastic foundation, we derived a bucking parameter that combines the effects of geometry and material properties to predict the transition between different periodic buckling modes and constructed a phase diagram to guide the design of periodic buckling tubes for bioinspired functional gel structures.
One of the pleasures of teaching is seeing how well your former students are doing in their new adventures. I ran into JHU ME alumni Bailey Hannon and Martha Whiting, BS 2015 at ARVO. I taught them both in Biosolids and Martha built an essential test fixture for my lab as an REU student. Both were presenting their research and Bailey gave a great talk on using machine learning to automatically count axons. Bailey is a 4th year PhD student in BME at Georgia Tech and Martha is a 3rd year MD student at U. of Maryland.
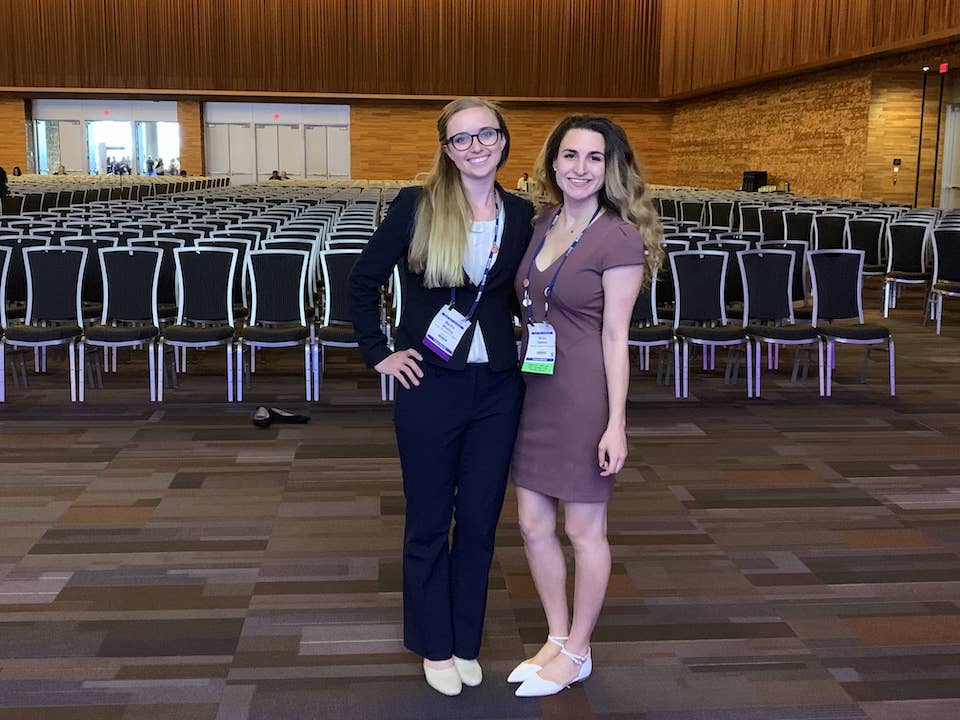
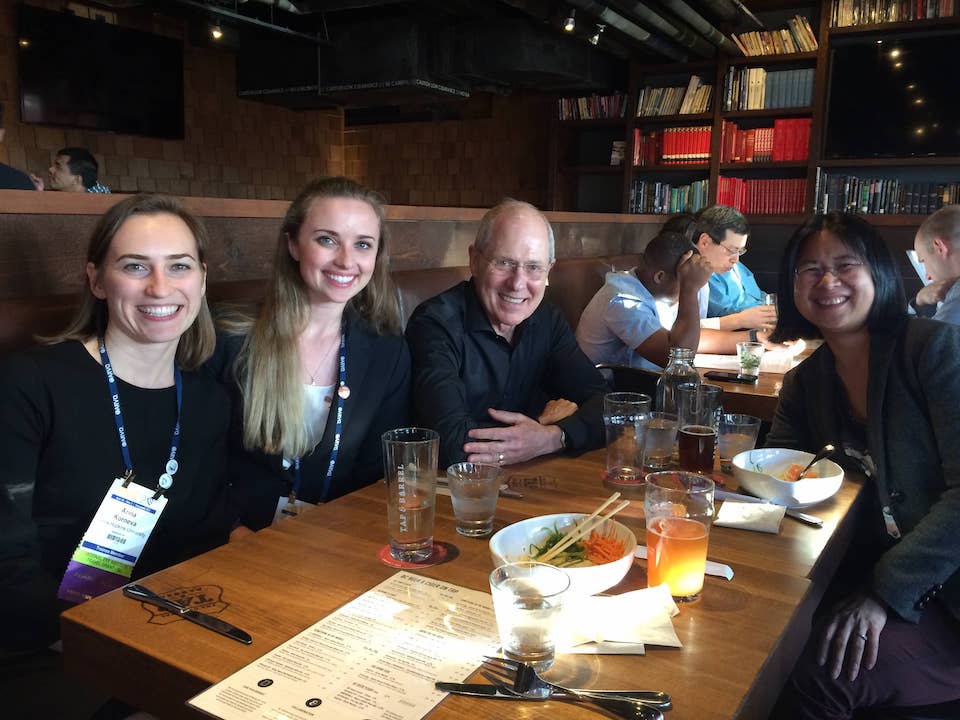
Congratulations to Arina Korneva for winning the Members In Training Poster Award Competition for the Glaucoma Section at the 2019 Annual Meeting at ARVO. Arina’s poster was titled “. Well done!
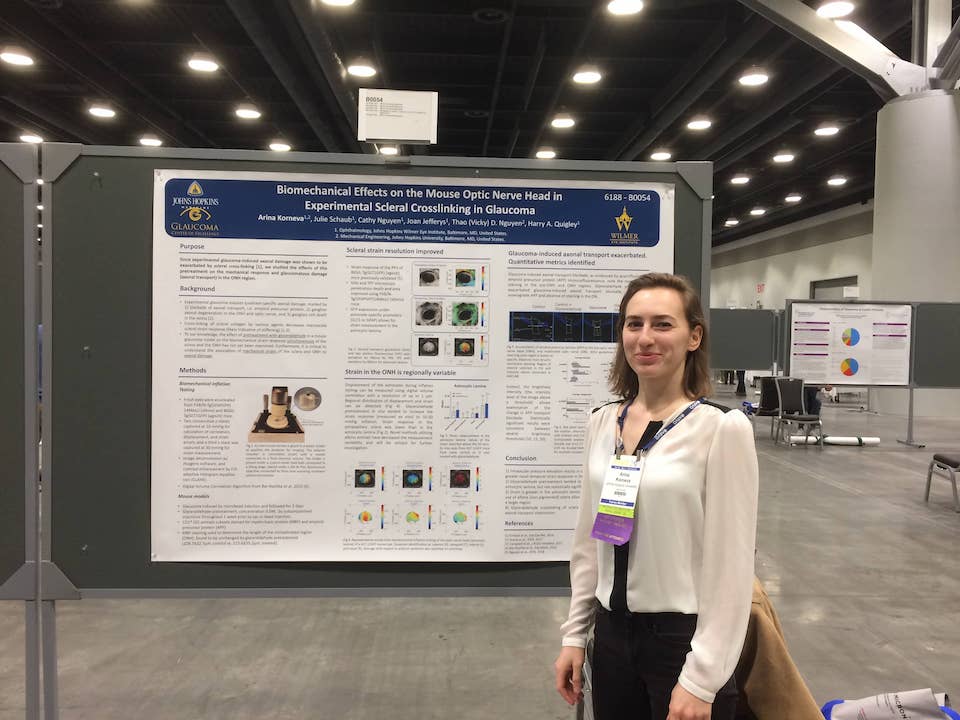
Congratulations to Dr. Michelle Chen for her successful dissertation defense, “Mechanochemistry of Collagen Network Remodeling in the Sclera“.
Abstract: The primary load-bearing tissue of the eye is the sclera, the eye’s white outer shell. It helps the eye maintain its shape by resisting intraocular pressure and protects the
delicate intraocular structures. The sclera is able to respond to mechanical changes in its environment by self- adapting, a process known as remodeling. This reaction, which alters the microstructure and/or properties of the tissue, is beneficial and clinically important but may also contribute to pathological diseases such as glaucoma and myopia when an imbalance in the remodeling process occurs. It is therefore important to understand the underlying mechanisms that drive the remodeling process, and how it affects tissue microstructure and its mechanical properties. This work focuses on two main parts of the connective tissue microstructure: collagen, which serves as the primary load-bearing component and dominates the anisotropic large-strain mechanical response of the tissue, and glycosaminoglycans (GAGs) which regulate the spacing between collagen fibrils and help determine tissue hydration. The objective of this work is to study the effect of remodeling on mechanical behavior of the posterior sclera. We investigate this through three case studies.
The first part of this work studies the mechanical role of GAGs in the human posterior sclera. Although alterations in mechanical properties and GAG content have been reported in glaucomatous eyes, it is unknown if the change in GAG content directly contributes to the observed changes in mechanical properties. Experimental protocols and novel analysis methods were developed for determining the inflation response of posterior human sclera shells, measured before and after enzymatic GAG degradation. It was shown that GAGs play a measurable role in altering the structure properties and mechanical behavior of the posterior human sclera, likely through their effects on hydration and their interactions with the collagen fibrils.
The second case study investigates how cyclic preconditioning of a collagenous substrate induces material and morphology property changes in collagen fibrils. Experimental studies have shown that repeated cyclic loading of an acellular collagen construct increased collagen stiffness, but did not significantly change fibril anisotropy. A model for the collagen fibril substrate was developed to show that the changes in the collagen anisotropy measured in experiments were insufficient to explain the measured increase in the stiffness of the collagen constructs with cyclic loading. The findings suggest that mechanical loading can induce changes in the stiffness and failure properties of the collagen fibril network through passive chemomechanical processes.
The last part of this work examines the effect of changes in the geometry of the sclera, collagen anisotropic structure, and material properties from glaucoma on the inflation response of the mouse sclera. An inverse finite element method was used to fit model parameters describing fibril properties to the average measured scleral edge displacements of glaucoma and normal mouse eyes. The findings show that changes in collagen fibril material properties are responsible for the observed stiffening effect in glaucoma mouse eyes. The observed structural changes associated with glaucoma did not meaningfully stiffen the mechanical response.
We celebrated the end of 2018 and beginning of 2019 with our annual get together. There was a Battle Ship and Mario Kart face-off between PhD students and postdoc and a 9 year old. Guess who won most of the time. There were also lots of food and a dessert bake off. Vicky Nguyen won with a almond cake, but Michelle Chen and Arina Korneva came in a very close second with a hazelnut cake and delicious brownie like cookies. Clearly everyone at the party was the winner because we all got to eat the desserts.
Congratulations to Dr. Jingkai Guo for his successful dissertation defense, “Modeling the Mechanical Behavior of Soft Active Materials“. Abstract below.
Soft active materials are capable of converting chemical or physical energy into mechanical work in response to various environmental stimuli such as pH, solvent, salt, heat, humidity, electric or magnetic field, and light. These materials are generally inexpensive, lightweight and their properties can be tailored based on requirements of applications. These attractive features give them great potentials in a wide range of applications, such as biomimetic systems inspired from living organisms. The objective of this work was to develop theoretical models that are capable of accurately describing the physical- mechanical behavior of soft active materials. A common set of methods that can be applied to the study of wide range of soft materials was developed. The methods covered different stages of the study, from material characterization to constitutive modeling, from simulation to experiments.
In this work, I focused on three kinds of soft active materials: dielectric elastomers, thermoresponsive gels and shape memory polymers. In the first part of this work, we investigated the temperature dependent viscoelastic behavior of dielectric elastomers and the effects of viscoelasticity on the electro- actuation behavior. We measured the viscoelastic relaxation spectrum of VHB 4905 and applied the results to a discrete multi-process viscoelastic model. The model generally showed good quantitative agreements with experimental measurements in terms of both pure-mechanical and electro-mechanical behaviors of the material. The model was able to qualitatively capture the dependence of the electric breakdown time on voltage and pre-stretch. In the second part of this work, we studied the thermoresponsive bilayer plates with soft and stiff segments that exhibit bidirectional and biaxial curving. We investigated the mechanism underlying the deformation modes through finite element simulation and explored the effects of geometry factors such as the aspect ratio and the segment spacing. In the final part of this work, we developed a constitutive model based on the effective temperature theory that is capable of describing strain hardening behavior in a thermodynamically consistent manner. The model incorporated two mechanisms: one represents the stretching and orientation of the polymer network, which leads to the development of a backstress; the other one represents the molecular reptation, which accounts for the temperature and rate dependence of strain hardening. The model was implemented into finite element programs and was applied to simulate the thermomechanical behavior of polycarbonate. All of the model parameters were determined through standard thermomechanical tests. The simulation results showed good agreements with experiments and the temperature dependence, strain rate dependence and strain state dependence of hardening were quantitatively captured.