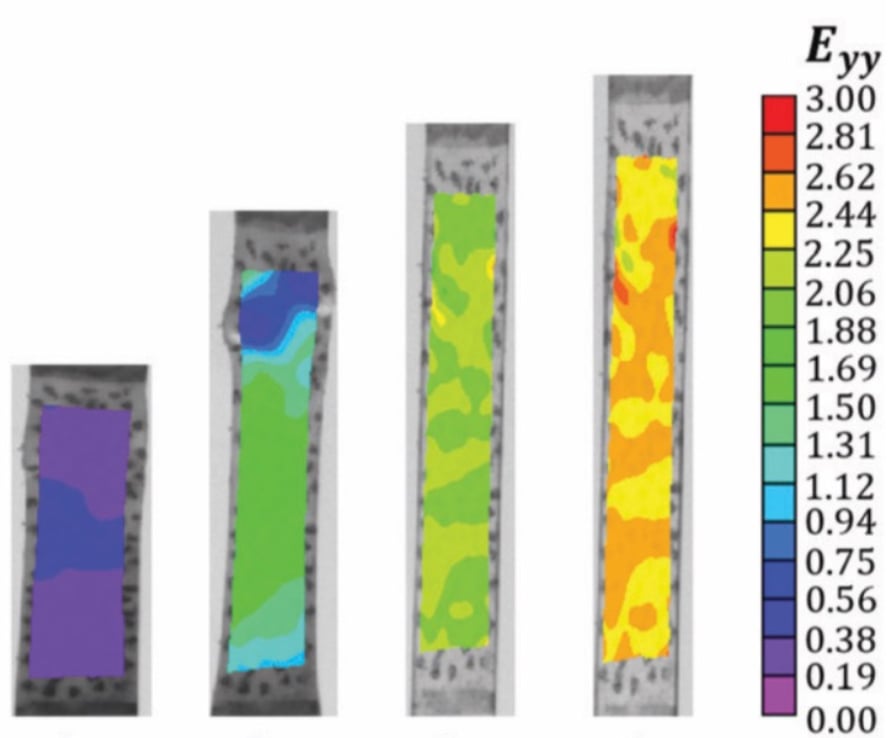
Liquid-crystalline elastomers (LCEs) are a class of soft stimuli-responsive materials composed of stiff mesogens bound to an elastomeric network of flexible polymer chains. The mesogens can order and disorder in response to an external stimulus, such as temperature and mechanical deformation. This allows LCEs to undergo reversible phase transitions between the polydomain, monodomain, and isotropic states. The motion of the mesogens relative to the polymer network also leads to unusual behavior, including large reversible actuation in response to temperature or light, soft-elasticity, and enhanced dissipation. The viscoelastic properties of LCEs can be exploited to design transformative materials and structures with extreme dissipation behavior.
We seek to develop a fundamental understanding of the relaxation mechanisms of main-chain LCEs to enable the design of extraordinary dissipation behaviors of LCE materials and structures over multiple length scales. We hypothesize that the enhanced dissipation behavior of LCEs arise from the coupling of the relaxation dynamics of the mesogens and polymer network, and depends on the mesogen order and chain alignment. Furthermore, the intrinsic dissipation behavior can be exploited in an architected design to produce structures with extreme damping and energy absorption.
Our research aims are:
- Characterize the anisotropic relaxation behavior of the mesogens and polymer network using wide-angle X-ray scattering and LCEs with tailored structures.
- Develop a nonlinear thermoviscoelasticity theory for LCEs.
- Design, fabricate and characterize architected LCE structures.
Figure 1: (a) The stress response of a polydomain main-chain LCE to a load-unload cycle (a) for 0.01/s and different temperatures ranging from the glass transition temperature Tg to the nematic-isotropic temperature Tni, and (b) for different strain rates at 20oC. The stress response of a monodomain main-chain LCE to a load-unload cycle applied (d) perpendicular and (e) parallel to the director. The stress response is highly rate-dependent with large hysteresis. The peak stress is rate-dependent, which suggests a viscous director rotation. Loading parallel to the director does not induce director rotation but still has significant hysteresis.
Figure 2: Contour of the axial strain field determined by incremental 3D digital image correlation (DIC) for uniaxial tension at 2 different strain rates for a polydomain LC. Rate-dependent mesogen relaxation produces a inhomogeneous strain field in the semi-soft stress region for the slower 0.0001/s strain rate. By comparison, the strain field for the faster 0.1/s strain rate is more homogeneous.
Figure 3: Nonlinear viscoelastic micropolar model for a mondomain LCEs, showing (a) rheological representation of the model, Rate-dependent uniaxial tension stress response for loading (b) perpendicular and (c) parallel to the director orientation comparing simulations and experiments.
Figure 4: (a) Finite element simulations of an LCE metamaterial, composed an array of bistable LCE beams constrained by rigid horizontal supports, under compression. The viscoelastic LCE beams buckle non uniformly, the layers to collapse sequential. When each layer buckles the other layers recovers before bundling again. (b) The individual buckling of the layers causes the force displacement curve to exhibit multiple peaks compared to the homogenous buckling case. (c) The difference in the viscous dissipation energy and stored elastic energy between the homogenous and inhomogeneous buckling case for different strain rates and number of layers. The cycle of compression and recovery increases the viscous dissipation energy which increases the total energy absorption.
Collaborators
- Chris Yakacki, Ph.D., Professor, Department of Mechanical Engineering, University of Colorado Denver
- Carl Frick, Ph.D., Professor, Department of Mechanical Engineering, Colorado School of Mines
- Sanjay Govindjee, Ph.D., Department of Civil and Environmental Engineering,
- Sung Hoon Kang, PhD., Assistant Professor, Department of Mechanical Engineering, Johns Hopkins University
- Aaron Forster, Ph.D. Staff Scientist. Security Technologies Group, National Institutes of Standard and Technology